Epigenetics: Beyond the Genome
Did you know that the choices we make every day can influence our genes without altering their sequence? This fascinating realm of biology, known as epigenetics, unveils a new layer of understanding about how our genes operate and how external factors can modify their expression.
Epigenetics refers to the study of heritable changes in gene expression that do not involve alterations to the underlying DNA sequence. Essentially, it is the science of how genes can be turned on or off and how this regulation impacts our health and disease states.
Understanding epigenetics is crucial because it offers insights into the complexity of human biology beyond the static DNA code. It reveals how lifestyle choices, environmental exposures, and even social experiences can leave lasting marks on our genome, influencing everything from development and aging to the susceptibility to diseases like cancer and diabetes.
Notable Labs is pioneering efforts in the field of epigenetics, focusing on how these changes can influence gene expression without altering the DNA sequence itself. Through its advanced Predictive Precision Medicine Platform (PPMP), Notable combines multi-dimensional biological assays and machine learning to simulate a patient's cancer treatment and predict their clinical response. This approach helps in identifying patients who are likely to respond to specific therapies, thus fast-tracking clinical development.
Notable’s work in epigenetics is aimed at understanding and manipulating the epigenome to enhance treatment outcomes. Their platform has demonstrated high predictive precision in several clinical validation studies with recognized academic centers. By focusing on epigenetic mechanisms, Notable aims to develop therapies that can more accurately target cancer cells and improve patient outcomes, particularly in cases where traditional genetic approaches may fall short.
These activities underscore Notable’s commitment to revolutionizing cancer treatment through epigenetic research, providing new insights and potential therapies that go beyond traditional genomic approaches
Understanding Epigenetics
Epigenetics is a branch of biology that explores how gene activity can be regulated by mechanisms other than changes in the DNA sequence itself. These changes can be influenced by various factors, including the environment, lifestyle, and even experiences.
Key Mechanisms
- DNA Methylation: This involves the addition of a methyl group to the DNA molecule, usually at cytosine bases. Methylation typically acts to repress gene transcription. It plays a crucial role in various biological processes, including development, genomic imprinting, and X-chromosome inactivation.
- Histone Modification: Histones are proteins around which DNA winds, forming a structure known as chromatin. The modification of histones through processes like acetylation, methylation, and phosphorylation can influence chromatin structure and gene expression. These modifications can either tighten or loosen the chromatin, thereby regulating access to the genetic information.
- Non-Coding RNA: Non-coding RNAs, such as microRNAs (miRNAs) and long non-coding RNAs (lncRNAs), play significant roles in the regulation of gene expression at the transcriptional and post-transcriptional levels. These RNAs can guide chromatin-modifying complexes to specific genomic locations or interfere with the translation of messenger RNA (mRNA) into proteins.
Historical Perspective
The field of epigenetics has a rich history that dates back to the early 20th century, but it has gained significant momentum in recent decades. Here are some key milestones:
- Conrad Waddington (1942): The term "epigenetics" was first coined by British biologist Conrad Waddington, who proposed that genes and their expression could be influenced by factors beyond the genetic code, leading to different phenotypic outcomes.
- DNA Methylation (1975): The discovery of DNA methylation as a key epigenetic mechanism was a significant breakthrough. Researchers like Adrian Bird and Arthur Riggs demonstrated how methylation could influence gene expression, paving the way for understanding epigenetic regulation.
- Histone Code Hypothesis (2000): The proposal of the histone code hypothesis by Brian Strahl and C. David Allis highlighted the complexity of histone modifications in gene regulation. This hypothesis suggested that the combination of various histone modifications could create a code that determines chromatin structure and gene activity.
- Human Epigenome Project (2003): Following the completion of the Human Genome Project, the Human Epigenome Project was initiated to map the epigenetic modifications across the entire genome. This project aimed to understand how these modifications contribute to human development, health, and disease.
These milestones have significantly advanced our understanding of how epigenetic mechanisms regulate gene expression and have opened new avenues for research and therapeutic interventions.
Mechanisms of Epigenetic Regulation
DNA Methylation
DNA methylation is one of the most well-studied epigenetic mechanisms. It involves the addition of a methyl group to the fifth carbon of the cytosine ring, forming 5-methylcytosine. This process is catalyzed by DNA methyltransferases (DNMTs) and typically occurs at CpG dinucleotides, regions where a cytosine nucleotide is followed by a guanine nucleotide in the linear sequence of bases.
Role in Gene Expression Regulation
DNA methylation plays a crucial role in regulating gene expression. When methyl groups are added to the promoter region of a gene, it can prevent the binding of transcription factors and other regulatory proteins, leading to gene silencing. This mechanism is essential for processes such as genomic imprinting, X-chromosome inactivation, and suppression of transposable elements. Abnormal DNA methylation patterns have been linked to various diseases, including cancer, where hypermethylation of tumor suppressor genes or hypomethylation of oncogenes can contribute to uncontrolled cell growth.
Histone Modification
Histones are the main protein components of chromatin, which package and order DNA into structural units called nucleosomes. Each nucleosome consists of DNA wrapped around a histone octamer, which includes two copies each of histone proteins H2A, H2B, H3, and H4. Histone modifications, such as acetylation, methylation, phosphorylation, and ubiquitination, occur on the histone tails and influence chromatin structure and gene expression.
Impact on Gene Accessibility and Expression
Histone modifications can either relax or compact chromatin structure, thereby regulating gene accessibility. For instance, acetylation of histone tails by histone acetyltransferases (HATs) neutralizes the positive charge on histones, reducing their affinity for the negatively charged DNA and resulting in a more relaxed chromatin structure that is accessible to transcription machinery. Conversely, histone deacetylases (HDACs) remove acetyl groups, leading to chromatin condensation and gene silencing. Similarly, methylation of histone H3 on lysine 4 (H3K4) is associated with active transcription, while methylation on lysine 27 (H3K27) is linked to gene repression.
Non-Coding RNA
Non-coding RNAs (ncRNAs) are RNA molecules that are not translated into proteins but play crucial roles in regulating gene expression. They can be broadly categorized into small non-coding RNAs (such as microRNAs) and long non-coding RNAs (lncRNAs).
Role in Gene Regulation
ncRNAs regulate gene expression at various levels, including chromatin remodeling, transcription, and post-transcriptional processing.
Examples and Functions
- MicroRNAs (miRNAs): These are short RNA molecules, typically 20-24 nucleotides long, that regulate gene expression by binding to complementary sequences on target mRNAs, usually resulting in gene silencing through mRNA degradation or inhibition of translation. For example, miR-21 is known to target and downregulate tumor suppressor genes, playing a role in cancer progression.
- Long Non-Coding RNAs (lncRNAs): These are longer RNA molecules, usually more than 200 nucleotides in length, involved in various regulatory functions. They can interact with chromatin-modifying complexes to alter chromatin states, serve as scaffolds for protein complexes, or act as molecular sponges to sequester other regulatory RNAs or proteins. An example is Xist, a lncRNA crucial for X-chromosome inactivation in females.
These mechanisms illustrate the intricate network of epigenetic regulation, highlighting how modifications beyond the DNA sequence can have profound effects on gene expression and cellular function. Understanding these processes opens up new possibilities for therapeutic interventions in diseases where epigenetic regulation is disrupted.
Impact on Health
Epigenetic changes can have a significant impact on health outcomes. They play a role in development, aging, and the body's response to environmental stimuli. For example, lifestyle factors such as diet, stress, and exercise can induce epigenetic changes that affect gene expression and, consequently, health.
- Diet: Nutrients and bioactive food components can influence epigenetic mechanisms. For instance, folate, a B vitamin, is crucial for the methylation process. A diet rich in folate can enhance DNA methylation and potentially protect against diseases like cancer.
- Stress: Chronic stress has been shown to alter epigenetic marks, particularly in genes involved in the stress response. These changes can affect mental health and contribute to conditions like depression and anxiety.
- Exercise: Physical activity can lead to beneficial epigenetic modifications, such as increased acetylation of histones associated with genes involved in muscle growth and metabolism. This can improve overall health and reduce the risk of chronic diseases.
Epigenetics and Disease
Epigenetic dysregulation is implicated in various diseases, including cancer, neurological disorders, and cardiovascular diseases. Specific examples illustrate how epigenetic changes contribute to disease:
- Cancer: Aberrant DNA methylation and histone modifications are hallmarks of cancer. For example, the hypermethylation of tumor suppressor genes like BRCA1 and the hypomethylation of oncogenes can lead to uncontrolled cell proliferation and tumor growth. In colorectal cancer, the CpG island methylator phenotype (CIMP) is characterized by widespread promoter hypermethylation, leading to the silencing of multiple tumor suppressor genes.
- Neurological Disorders: Epigenetic mechanisms are crucial for brain development and function. Alterations in DNA methylation and histone modifications are associated with neurodevelopmental disorders such as autism spectrum disorders (ASD) and neurodegenerative diseases like Alzheimer's disease. For instance, reduced expression of MeCP2, a protein involved in reading DNA methylation marks, is linked to Rett syndrome, a severe neurological disorder.
- Cardiovascular Diseases: Epigenetic modifications play a role in the development of cardiovascular diseases. Hypermethylation of genes involved in lipid metabolism and inflammation can contribute to atherosclerosis. In hypertension, altered expression of genes regulated by histone modifications can affect vascular function and blood pressure regulation.
Case Studies
- Cancer: The role of epigenetics in cancer is exemplified by the case of acute promyelocytic leukemia (APL). Treatment with all-trans retinoic acid (ATRA) and arsenic trioxide (ATO) targets the epigenetic silencing of the PML-RARA fusion gene, leading to differentiation of the leukemic cells and remission in patients.
- Neurological Disorders: In Alzheimer's disease, the hypermethylation of the gene encoding the amyloid precursor protein (APP) has been observed, which is associated with the accumulation of amyloid plaques in the brain, a hallmark of the disease.
- Cardiovascular Diseases: Studies have shown that patients with coronary artery disease have differential DNA methylation patterns in genes related to lipid metabolism and inflammation, which can serve as biomarkers for disease diagnosis and prognosis.
Understanding the role of epigenetics in health and disease not only enhances our knowledge of disease mechanisms but also opens new avenues for therapeutic interventions. Epigenetic therapies, such as DNMT inhibitors and HDAC inhibitors, are being developed and tested in clinical trials, offering hope for treating diseases linked to epigenetic dysregulation.
Epigenetic Therapies and Precision Medicine
Current Therapies
Epigenetic therapies aim to reverse abnormal epigenetic modifications and restore normal gene function. Several drugs targeting these mechanisms have been developed and approved for clinical use, particularly in cancer treatment.
- DNA Methyltransferase Inhibitors (DNMT inhibitors): These drugs, such as azacitidine (Vidaza) and decitabine (Dacogen), inhibit DNA methyltransferases, leading to the reactivation of silenced tumor suppressor genes. They are used to treat certain types of leukemia and myelodysplastic syndromes. Clinical trials have shown that these drugs can induce remissions and improve survival rates in patients.
- Histone Deacetylase Inhibitors (HDAC inhibitors): Drugs like vorinostat (Zolinza) and romidepsin (Istodax) inhibit histone deacetylases, leading to an open chromatin structure and reactivation of suppressed genes. These inhibitors are used to treat cutaneous T-cell lymphoma and other malignancies. They have shown effectiveness in inducing cancer cell death, reducing tumor growth, and enhancing the effects of other cancer therapies.
Future Prospects
The potential for new therapies targeting epigenetic changes is vast. Researchers are exploring various strategies to develop more specific and effective epigenetic drugs.
- Targeting Histone Methyltransferases and Demethylases: New drugs are being developed to target enzymes involved in adding or removing methyl groups from histones. These drugs aim to specifically modify histone marks associated with disease states, offering a more targeted approach to therapy.
- CRISPR-based Epigenome Editing: The CRISPR-Cas9 system is being adapted to target and modify specific epigenetic marks. This technology allows for precise editing of DNA methylation and histone modifications, providing a powerful tool for studying epigenetic regulation and developing personalized therapies.
Role of Epigenetics in the Future of Precision Medicine
Epigenetics holds great promise for the future of precision medicine, where treatments are tailored to individual patients based on their genetic and epigenetic profiles. By understanding a patient's unique epigenetic landscape, clinicians can develop more effective and personalized treatment plans.
- Biomarkers for Disease Diagnosis and Prognosis: Epigenetic markers can serve as valuable biomarkers for early disease detection, monitoring disease progression, and predicting treatment response. For example, DNA methylation patterns in specific genes can help diagnose cancer at an early stage and guide treatment decisions.
- Personalized Epigenetic Therapies: As our knowledge of the epigenome expands, personalized epigenetic therapies can be developed to target the specific epigenetic abnormalities present in an individual's disease. This approach aims to enhance treatment efficacy and reduce side effects.
In conclusion, the field of epigenetics is transforming our understanding of gene regulation and its impact on health and disease. Epigenetic therapies offer promising new avenues for treating various diseases, and the integration of epigenetics into precision medicine has the potential to revolutionize healthcare by providing more personalized and effective treatments. As research continues to uncover the complexities of the epigenome, the future of epigenetics holds exciting possibilities for improving human health and well-being.
Ethical and Social Implications
Ethical Considerations
Epigenetic research and therapies bring about several ethical dilemmas that must be addressed to ensure responsible scientific advancement and patient care.
- Informed Consent: As epigenetic studies often involve sensitive personal data, obtaining informed consent from participants is crucial. Participants should be fully aware of the nature of the research, potential risks, and how their data will be used and protected.
- Privacy and Genetic Discrimination: The information obtained from epigenetic research can reveal a lot about an individual's predisposition to certain diseases and their biological responses to environmental factors. This raises concerns about privacy and the potential misuse of this information, such as genetic discrimination by employers or insurance companies. Protecting individuals' rights and ensuring confidentiality is paramount.
- Intergenerational Effects: Epigenetic changes can be heritable, affecting not only individuals but also their offspring. Ethical considerations must be taken into account when evaluating the long-term implications of epigenetic modifications and therapies, particularly in germline editing.
Social Implications
The growing understanding of epigenetics has significant implications for public health policies and societal views on health and lifestyle choices.
- Public Health Policies: Knowledge of epigenetic mechanisms can inform public health initiatives aimed at disease prevention and health promotion. For example, policies encouraging healthy diets, stress management, and regular exercise can be reinforced by the understanding that these lifestyle choices have epigenetic impacts that can prevent or mitigate diseases.
- Societal Views on Health and Lifestyle Choices: The realization that our behaviors and environment can influence our genes may lead to a shift in societal perspectives on health and responsibility. This knowledge could promote a more proactive approach to health, emphasizing the importance of lifestyle choices in disease prevention and overall well-being.
- Education and Awareness: Raising public awareness about epigenetics can empower individuals to make informed decisions about their health. Educational programs can help demystify the science of epigenetics and highlight its relevance to everyday life, encouraging healthier behaviors and greater engagement with preventive health measures.
In summary, while epigenetic research holds great promise for advancing our understanding of gene regulation and developing new therapies, it also presents ethical and social challenges that must be carefully navigated. By addressing these issues and integrating ethical considerations into research and clinical practices, we can harness the potential of epigenetics to improve health outcomes while safeguarding individual rights and societal values.
Summary of Key Points:
In this blog, we delved into the fascinating world of epigenetics and its profound implications for health, disease, and medicine. Here are the key takeaways:
- Epigenetics Overview: Epigenetics involves heritable changes in gene expression that do not alter the DNA sequence. It reveals how our environment and lifestyle choices can affect gene activity and influence health outcomes.
- Mechanisms of Epigenetic Regulation: The main mechanisms include DNA methylation, histone modification, and non-coding RNA. These processes regulate gene accessibility and expression, impacting various biological functions and disease states.
- Health and Disease: Epigenetic changes play a crucial role in development, aging, and disease. Factors like diet, stress, and exercise can induce beneficial or harmful epigenetic modifications. Dysregulation of these processes is linked to diseases such as cancer, neurological disorders, and cardiovascular diseases.
- Epigenetic Therapies: Current therapies, including DNMT inhibitors and HDAC inhibitors, aim to reverse abnormal epigenetic modifications. Future prospects include targeted therapies using histone methyltransferase inhibitors and CRISPR-based epigenome editing.
- Ethical and Social Implications: Ethical considerations include informed consent, privacy, and genetic discrimination. Social implications involve the impact on public health policies and societal views on health and lifestyle choices.
The field of epigenetics is poised to revolutionize medicine by offering deeper insights into gene regulation and new therapeutic avenues. As research advances, we can expect:
- Enhanced Disease Prevention and Treatment: By understanding individual epigenetic profiles, personalized therapies can be developed to target specific epigenetic abnormalities, improving treatment efficacy and reducing side effects.
- Integration with Precision Medicine: Epigenetics will play a pivotal role in precision medicine, enabling more accurate disease diagnosis, monitoring, and tailored treatment plans based on an individual's unique epigenetic landscape.
- Continued Ethical Considerations: Ongoing dialogue and ethical frameworks will be essential to navigate the complexities of epigenetic research and its applications, ensuring that advancements benefit society while protecting individual rights.
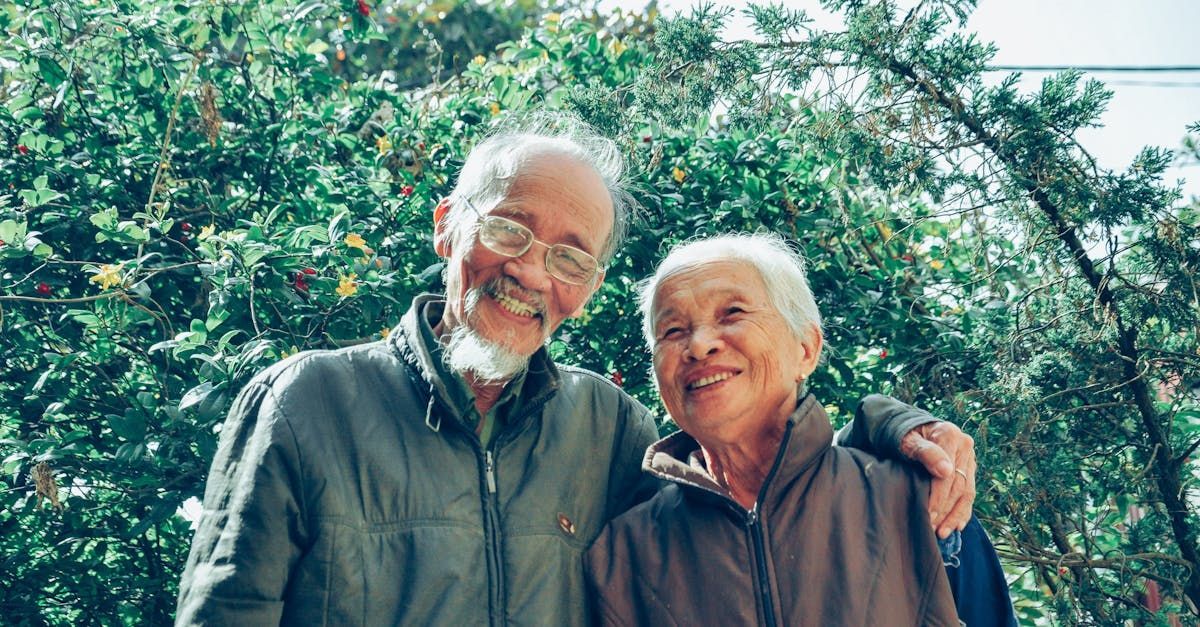
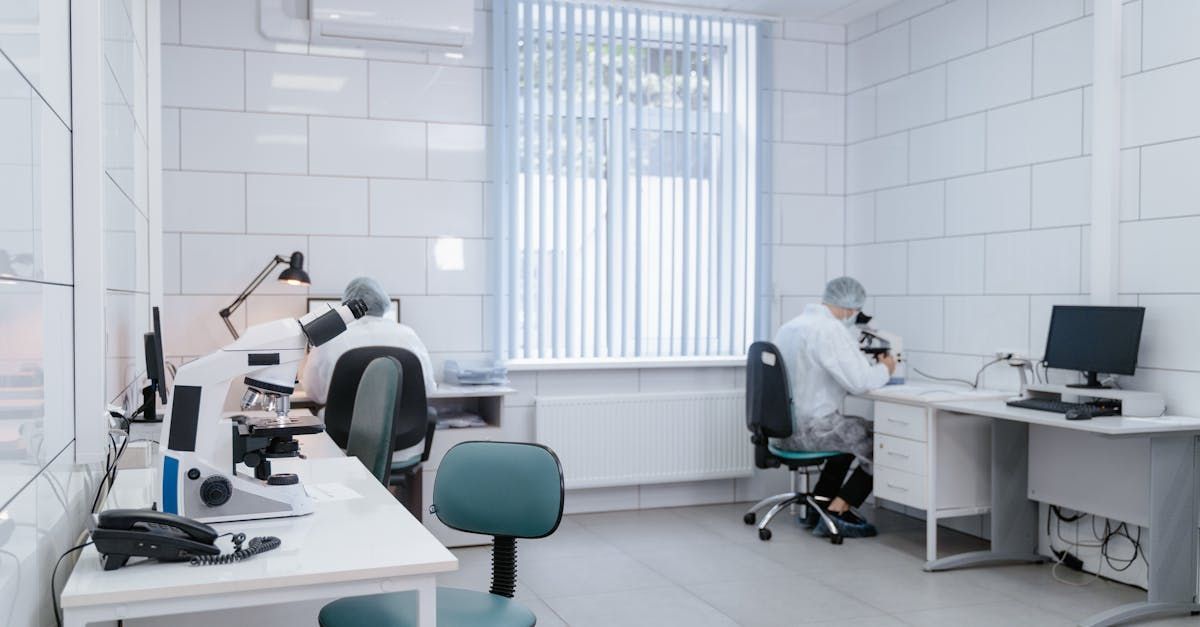